When it comes to exploration geology, age matters! In this article, we’ll discuss how rocks are dated, why it matters, and how geochronology is making waves in South African gold exploration.
What Is Geochronology?
The geologic timescale is daunting: even the most mundane rocks have a unique history stretching back millions, if not billions, of years. While relative timing between rocks can often be determined through intuitive principles (like cross-cutting relationships) giving rocks an absolute age is much trickier. A granite that is a few million years old may look very similar to a granite that’s a few billion years old. This is where geochronology–the scientific process of dating rocks–comes in.
Finding absolute dates of rocks is often the work of academics, but it can also be a powerful tool to assist mineral exploration. It allows geologists to make connections between mineralization and wider tectonic events such as specific episodes of volcanism or mountain-building. In turn, this facilitates the development of accurate deposit models to guide exploration efforts. Knowing when a deposit formed is often key to understanding how it formed, which in turn helps predict where ore may be found.
Many types of deposits are strongly associated with certain time periods. Looking for porphyry copper? Most of it is found in or near granites younger than about 65 Ma. Unconformity uranium? You won’t find it in rocks younger than 1.5 Ga. On the finer scale, it predicts if old ore is likely to be deformed, crosscut, or consumed by younger geological events. To put it simply: if you don’t know how old your deposit is, you don’t fully understand it.
How Does Geochronology Work?
Geochronology uses the law of radioactive decay to determine how old a rock is and put geologic history into definitive context. A radioactive element is one with an unstable nucleus–that is, the element’s nuclear loses energy and weight over time. This process is called radioactive decay and forms isotopes. Isotopes are variations of a specific element that have a different number of neutrons (and weight) due to radioactive decay.
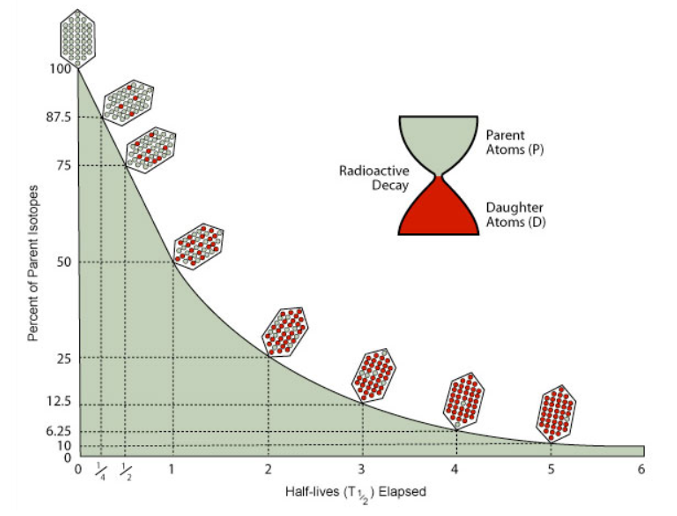
The decay of a radioactive isotope is governed by its half-life: the time it takes for half of the radioactive parent isotope to decay to its stable daughter isotope. The ratio between parent and daughter steadily evolves over time. Initially the ratio is 1:0, after one half-life it’s 1:1, after two half-lives it’s 1:3, after three half-lives it’s 1:7 and so on. If the length of the half-life is known, then measuring the current ratio between parent and daughter isotopes allows the absolute age of the rock or mineral to be determined. These ages are typically reported in millions of years (abbreviated as Ma) or billions of years (Ga) and with a corresponding error. Errors tend to become larger as age increases.
Geochronology Methods
Geochronology can be applied to a variety of parent/daughter systems and can be conducted by many analytical methods. While others exist, the systems and methods most commonly applied to ore deposits are summarized below.
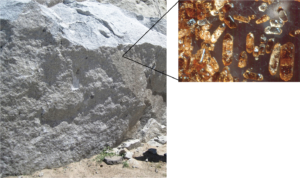
The Uranium-Lead (U-Pb) System
In this system, radioactive uranium decays to stable lead. The U-Pb system is the most common method of geochronology for one reason: zircons. These common igneous minerals nearly always contain enough U to be dated, and their extreme durability allows them to survive metamorphism and erosion, so they are often found in metamorphic and sedimentary rocks as well. It’s no exaggeration to say we’d know far less about geology as a whole it wasn’t for these little heroes of geochronology. Many other minerals, notably uraninite and monazite, may also be dated with the U-Pb method. Thorium, a radioactive element common in many rare Earth element deposits, also decays to lead, enabling thorium minerals to be dated via similar methods.
The Rhenium-Osmium (Re-Os) System
Although Re is one of the rarest elements on Earth, its decay to Os makes it particularly useful for dating ore deposits. Extremely trace amounts of Re are often present in sulfide minerals, such as pyrite and molybdenite, allowing these minerals to be directly dated. Since sulfides are the main ore minerals in most base metal deposits, this technique has found wide application, particularly to porphyry deposits.
The Potassium-Argon/Argon-Argon (K-Ar/Ar-Ar) System
While K is an extremely common element in rock-forming minerals, it is also weakly radioactive, and its decay to Ar allows minerals such as potassium feldspar and sericite to be dated. These common gangue minerals are formed by potassic and/or phyllic alteration in many deposits, and their ages should, in theory, reflect the age of the deposit. Argon, however, being a gas, may escape after its formation, and thus Ar dates may reflect geological events long after ore deposition. Great care must be taken when interpreting K-Ar and Ar-Ar data.
Instruments
SIMS/SHRIMP
Secondary Ion Mass Spectrometry (SIMS) and its beefed-up cousin Sensitive High-Resolution Ion MicroProbe (SHRIMP) use an ion beam to dislodge secondary ions from the sample, which are then magnetically directed into a mass spectrometer for analysis. This technique has the advantage of allowing very precise targeting of features as small as zoning within zircons, making it the preferred method for U-Pb dating.

TIMS
In Thermal Ionization Mass Spectrometry (TIMS) a sample (usually a whole mineral grain) is liquified and heated to generate ions, which are then analyzed in a mass spectrometer. This technique is known for its ability to analyze trace elements at concentrations other methods struggle to detect. This sensitivity makes it ideal for Re-Os dating, and allows superior accuracy in measured ages.
LA-ICPMS
Laser Ablation-Inductively Coupled Plasma Mass Spectrometry (mercifully shortened to LA-ICPMS) uses a laser to blast ions out of sample for analysis in a mass spectrometer. This method is something of a jack-of-all-trades: it’s very versatile, but generally can’t quite match the precision of SHRIMP or TIMS.
Argon Techniques
Argon-Argon dating requires samples of crushed rocks or minerals to be irradiated in a nuclear reactor before being heated to liberate Ar ions which are then measured in a mass spectrometer. K-Ar dating does not require irradiation but is considered more vulnerable to contamination and inaccurate ages.
How Is Geochronology Used In Mining?
As you may have guessed from the above, geochronology is complex and requires specialized and expensive instrumentation as well as qualified personnel, which mining companies generally lack access to. For this reason, many companies get their dates from previously published academic or government sources. If a company wishes to obtain their own dates, they may contract geochronology to a lab, or even partner with an outside institution or corporation as part of a larger study.
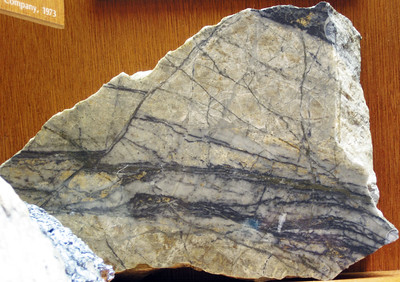
For an example of how geochronology is used, let’s look at the porphyry copper deposits around Orange Hill in Eastern Alaska. LA-ICPMS U-Pb dating of zircons dates granites in the area to 118-113 Ma. Re-Os dating of molybdenite from ore-bearing veins yielded ages of 115.23 ± 0.46 and 114.86 ± 0.47 Ma. This indicates that ore deposition in the area is linked to the formation of these granites. Furthermore, these ages are nearly identical to the 114.8 ± 1.0 Ma U-Pb and 113.3 ± 1.3 Ma Ar/Ar dates from two nearby copper-gold deposits. Taken as a whole, this indicates all three of these deposits share a similar origin, suggesting that ~110-120 Ma granites in the area may be highly prospective for copper-gold-molybdenum mineralization. Companies can use this geochronology data to narrow their exploration focus on the most promising areas.
South Africa’s Golden Dilemma
Gold mining has been a pillar of South Africa’s economy for well over a century–hardly surprising considering it hosts the Witwatersrand Basin, the world’s richest gold province. What is surprising is that despite 130 years of mining, no one can agree on how these deposits formed. The debate, arguably the most contentious issue in the history of economic geology, boils down to this: the evidence is ambiguous between placer/synsedimentary or hydrothermal deposition.
The question is anything but academic. South Africa’s gold production has been falling for decades as reserves dwindle, and no one can seem to find any new big deposits. Since placers only form in river gravels and conglomerates, their ancient equivalents, the placer deposit theory implies that spending money exploring outside of these conglomerates would be nothing but a waste. Hydrothermal deposits, on the other hand, may form in any type of rock. If this theory is correct the next multi-billion-dollar deposit may well await discovery in rocks that have remained underexplored.
This is where geochronology comes in. If the deposits are placer-style, the gold would have to be older than the host conglomerates (the age of which is known through SHRIMP U-Pb dating of zircons). If the deposits are hydrothermal, the gold would have to be younger than the conglomerates.
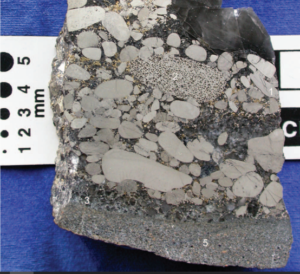
While gold itself can’t be dated, a controversial attempt to apply TIMS Re-Os dating to trace impurities within the gold yielded an age of ~3.0 Ga. This age is old enough to seemingly prove the placer theory correct. The validity of this age, however, has been heavily challenged on technical grounds. While the Witwatersrand is famous for gold, variable and occasionally economically interesting amounts of U in the form of uraninite are also present in many deposits. Recent SIMS U-Pb dating of this uraninite found it to be as old as 3186 ± 19 Ma, again strongly supporting the placer model.
While the debate isn’t over yet (there’s enough wiggle room in the hydrothermal model to prevent it being ruled out entirely), geochronology tips the scales in favour of placer models. It’s probably not a coincidence no major deposits have been found outside the conglomerates.
Geochronology can be complex and difficult to obtain, but when used properly it may yield a great deal of knowledge about ore deposition. Although this knowledge does not guarantee success in exploration and mining, it certainly makes it more likely.
Further Reading
- Geochronology and Nd-Sr-Pb isotopic compositions of Early Cretaceous intrusions and associated porphyry Cu deposits in eastern Alaska (scientific article – pdf)
- Evidence of a reducing Archean atmosphere from 3.2 Ga Witwatersrand Basin uraninite (scientific article)
Subscribe for Email Updates