Introduction
This article is the second in a series about Mineral Systems. The first, an Introduction to the Mineral Systems Method, is available here. In this second article, we present the other critical elements of Mineral Systems followed by examples of their applications for different types of ore deposits.
Fertility
The link between mineralization in specific tectonic settings is well-documented. Yet, economic mineralization within these settings is only occasional. For example, porphyry deposits occur in subduction-related magmatic arcs – however, not all subduction-related magmatic arcs will be mineralized. Therefore, ore-forming events must be controlled by an additional factor – the terrane’s fertility. The term fertility reflects how metal-endowed a specific region or geological time is. The main factors influencing fertility include early Earth evolution, crustal enrichment, large-scale geodynamics, and to a minor extent paleolatitude (i.e., the latitude of a place at the time of its formation measured based on the continental drift and movement of the earth’s magnetic poles).
The abundance of metals, and their mobility, is controlled by the secular differentiation of the Earth’s chemical reservoirs (e.g. lithosphere, hydrosphere, biosphere, and atmosphere) through time. For example, a variety of evidence in the geological record suggests the Earth is cooling. This is illustrated by the temporal constrain of magmatic deposits such as komatiite-hosted nickel deposits, which mostly existent at around 2.7 million years ago, with no occurrence after 1.9 million years ago. Komatiite is a type of mantle-derived volcanic rock that crystallizes from lava with > 18% weight of magnesium oxide content. The temperatures required to melt magmas of komatiitic composition (1600°C) are much higher than modern volcanic rocks (≤ 1250°C).
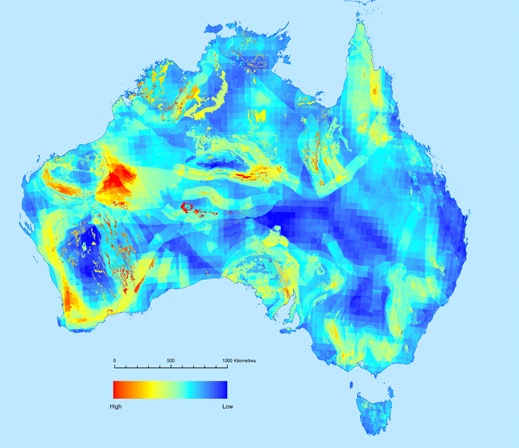
Drastic changes in the oxygen content of the atmosphere are recorded throughout the Earth’s evolution. The end of Archean (4.0-2.5 Ga) and Neoproterozoic (1.0-0.5 Ga) times is marked by an abrupt rise in atmospheric oxygen content. These oxygenation events had a major impact on the chemical stability of metals, ore minerals, and potential ligands (ions or molecules attached to metals) that transport them. One consequence was the formation of organic-rich shales which are optimal source rocks for the formation of Zn-Pb MVT-style deposits (Mississippi valley-type deposits, i.e. stratabound, often stratiform ores formed after the replacement of primary sedimentary rocks, particularly carbonate rocks).
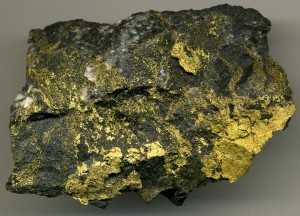
Some regions of the lithosphere are conspicuously more metal-endowed than others. This is exemplified by the anomalous enrichment of gold in greenstone belt sequences in parts of the North and South American Cordillera. Fertile areas typically contain deposits of a single commodity in several different styles of mineralization. Anomalous metal endowment potentially results from metasomatic enrichment of the upper mantle, however this is not well understood.
The cooling recorded throughout the Earth’s evolution influenced the mechanisms by which energy was transferred. It is believed that the early Earth was composed of a stagnant lithosphere with plume-driven tectonics that eventually transitioned to subduction and plate tectonics. The evolution of plate tectonics resulted in supercontinent cycles which cause periodic large-scale changes in geodynamic setting. The association of mineral deposits and specific tectonic regimes during these cycles is recognized for several ore deposit types. This is illustrated by the link between mafic-hosted nickel sulfide deposits and peaks in supercontinent assembly and the correlation of orogenic gold deposits with the end of accretionary events and supercontinent formation.
Paleolatitude is only critical for deposit types that require high-salinity fluids. That is because areas near the equator tend to have higher evaporation rates favorable to the formation of evaporites and residual brines. These salty fluids are critical to metal deposition, e.g. BIF-hosted Fe deposits and sediment-hosted uranium systems.
Preservation Potential of the Primary Depositional Zone

In addition to the factors that facilitate the formation of a deposit, geologic settings favorable to the preservation of mineralization through time must also be present (Figure 3). Most metal systems are deposited in the upper portion of the Earth’s crust, roughly delimited as the upper 10km. Depending on the type of mineral deposit, the preservation potential is different. That is partially explained by the tectonic settings at which these deposits are formed. In this context, deposits formed in elevated areas are more erosion-prone (e.g. porphyries) and tend to have a lower preservation potential than deposits formed in rifts (e.g. VMS – volcanogenic massive sulfide deposits) or at depth during late stages of an orogeny (e.g. orogenic gold deposits).
Investment in Exploration Targeting and Mineral Systems: What Do We Do Next?
In the past decades, we have been experiencing a decrease in discovery rates even with increasing exploration budgets. This may have been prompted by the various challenges associated with the way we have been doing regional exploration – by searching for deposit analogues. Alternatively, a solid mineral exploration workflow is proposed by the mineral system approach for targeting ore deposits. It can be applied by a broad understanding of the key processes that lead to the formation of mineralization. The multidisciplinary approach of mineral systems requires the consideration and integration of different datasets to evaluate an area. This promotes predictive criteria to narrow exploration areas and focus in prospective areas. Prospectivity maps are a key starting point when using the mineral systems approach (Figure 4).
The data integration promoted by a mineral systems perspective helps constrain the favorable ingredients that go into making a new deposit and predict potential time and place for undiscovered deposits. Maps showing the compilation of datasets across large continental areas can provide a window into the geological evolution of the crust and help understand the geological history of the area, therefore its potential for mineral resources. Thus, data- and knowledge-driven mineral prospectivity maps are excellent guides for greenfields exploration programs.
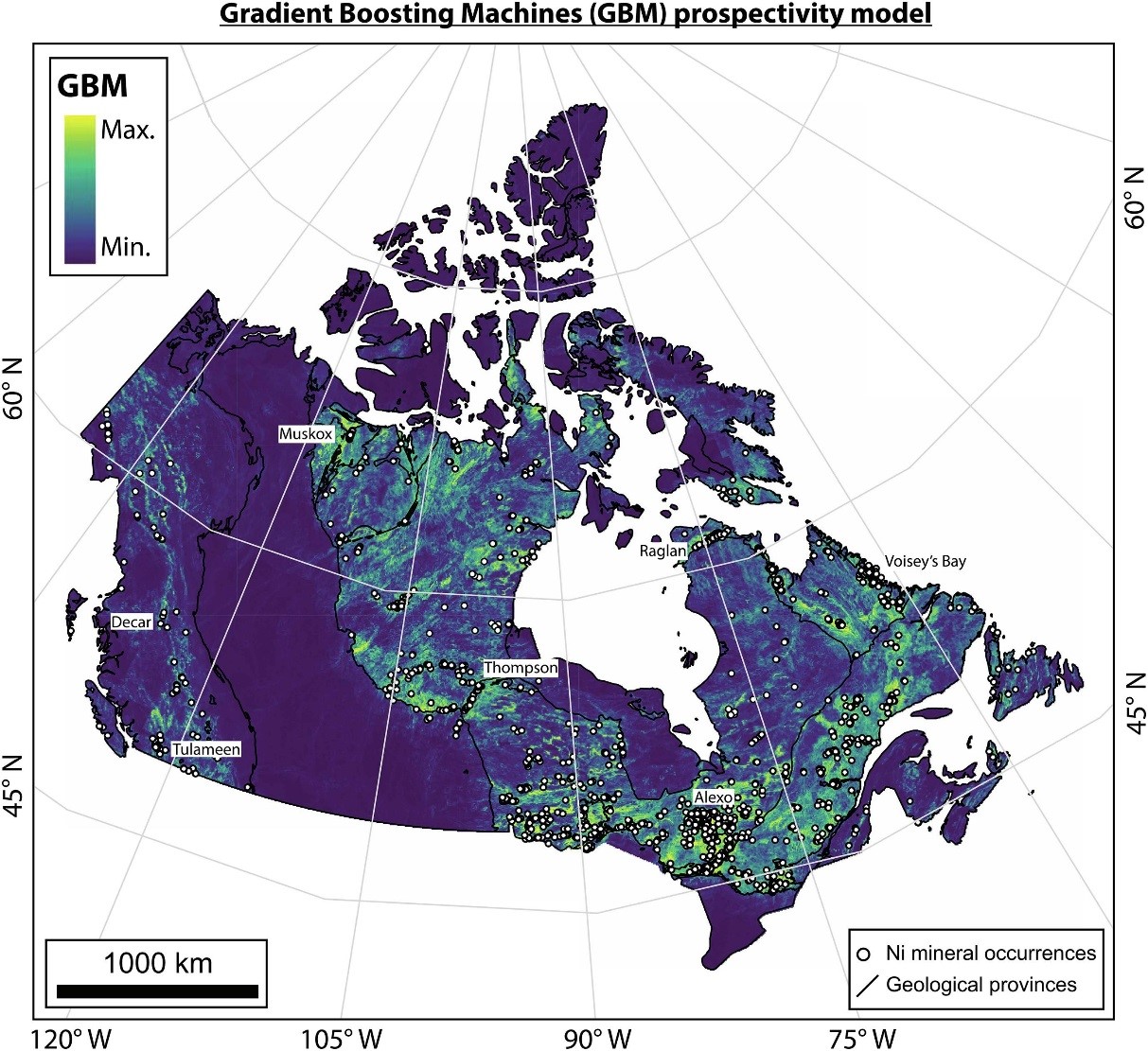