Outline
As the world shifts to a green economy, the rush to get commodities that can power this transition gains momentum. One commodity is set to play an essential role in developing alternative energy sources: lithium. This article is the second in a series about lithium deposits. The first, an overview of ‘hard rock’ lithium deposits, is available here.
Introduction
Lithium is a soft, silvery metal with highly reactive and flammable properties. Under standard temperature and pressure conditions, lithium is the lightest metal and the lightest solid element. Most lithium demand is driven by the battery industry.
Lithium Brine Deposits
Lithium-bearing brines are deposits of saline groundwater enriched in dissolved lithium that form in a closed-basin system. The saline accumulations that characterize brine deposits are common in nature, but only a few places in the world have the geological settings and arid climate that allow economic extraction of lithium. Geologic conditions for lithium brine deposits include closed basins and heat flow from hot springs or young volcanoes. The three main types of lithium brine deposits are continental, geothermal, and oil field.
Continental
Continental deposits, also known as salars or salt lakes/flats, are the most common form of lithium brine deposits. They consist of saline water concentrated in sandy areas with geothermal activity. Continental deposits in Bolivia, Argentina, and Chile represent approximately 66% of global lithium resources. Most of the world’s lithium production is in a region of the Andes known as the “Lithium Triangle” (Figure 1).
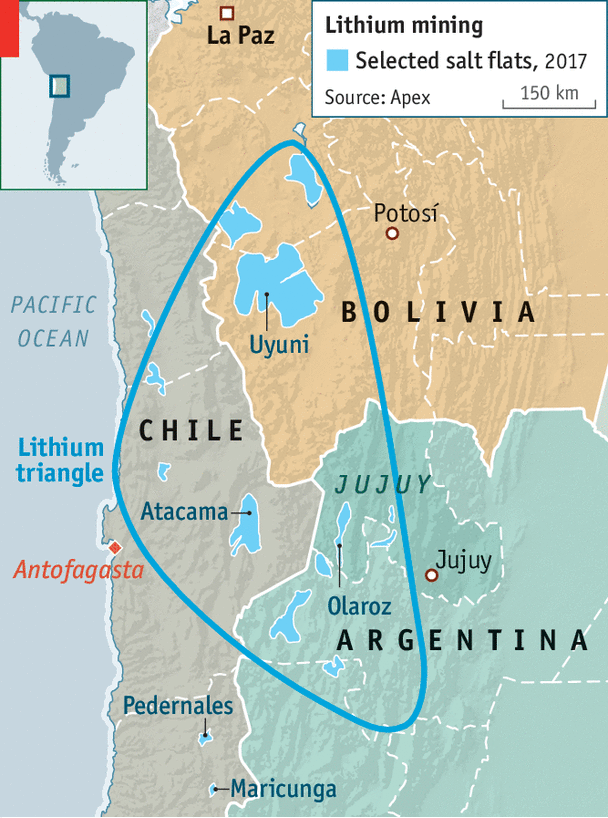
With 50-70% of known world lithium reserves, the Salar de Uyuni in Bolivia is the world’s largest lithium deposit. Yet, since the Bolivian government has control over the natural resources of the country, commercial exploitation of the Salar de Uyuni remains unclear. Added complications include the region’s low evaporation rates – 40% of that at Atacama – and the magnesium-rich nature of the deposits.
Another famous example of a continental lithium brine deposit in the Lithium Triangle is the Salar the Atacama, Chile, which has an estimated resource of 6.3 million MT at an approximate concentration of 0.14 % lithium. Chile-based Sociedad Quimica y Minera de Chile S.A. (NYSE:SQM) and US-based Albemarle (NYSE:ALB), two of the top lithium producers in the world, operate in the Salar de Atacama (Figure 2).
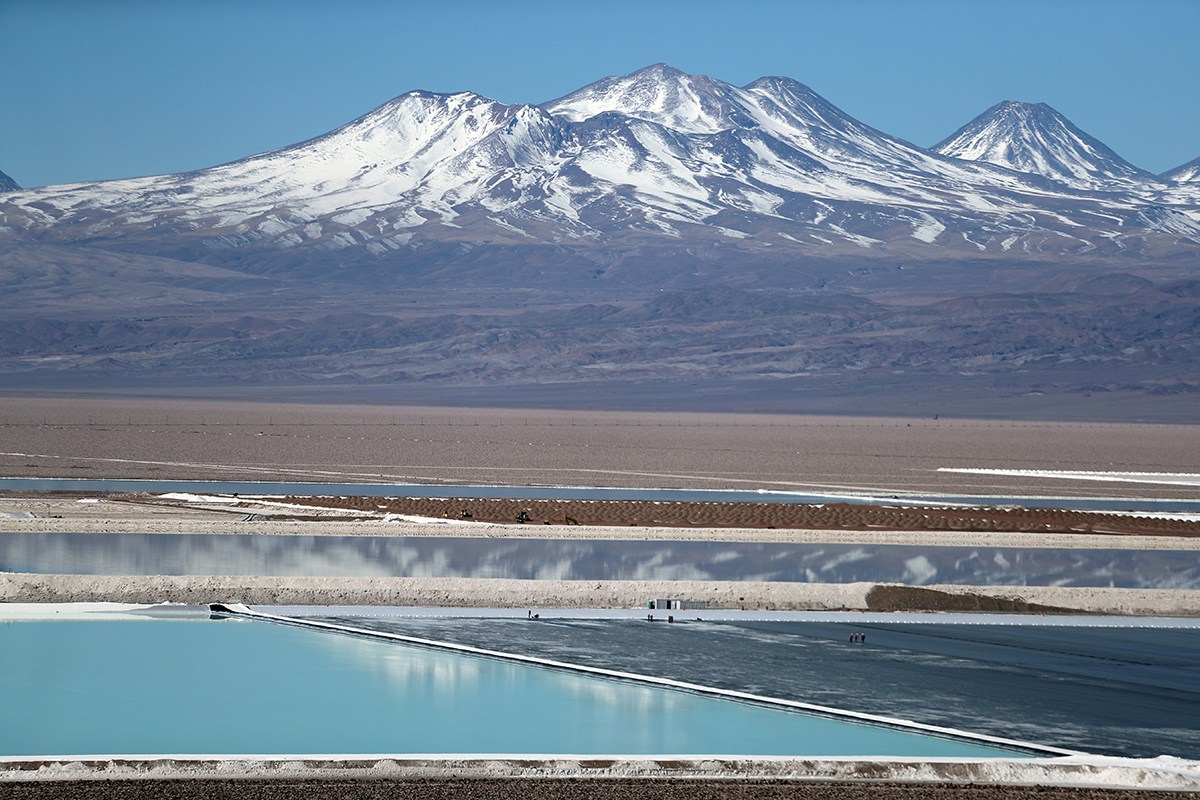
Located next to the only lithium brine operation in North America, Albemarle’s Silver Peak mine, Clayton Valley is a lithium-bearing claystone deposit owned by the Canada-based Cypress Development Corp. (TSX.V:CYP, OTC:CYDVF). The company is developing a pilot plant program following a recently published prefeasibility study for the Clayton Valley project (Figure 3).

Geothermal
Another important source of lithium includes geothermal deposits, which make up around 3% of known lithium resources in the world. These deposits originated in geothermal fields that form when hot water is pumped from underground reservoirs through faults and cracks within rock. Heat produced deep in the Earth is generated by the breakdown of radioactive isotopes (variants of a chemical element with extra energy in their core that make them unstable). Geothermal power is a clean, reliable (unlike solar or wind energy, it does not depend on weather conditions), and sustainable source of energy that has been used since ancient times for heating homes and cooking. Even so, it has been historically limited to areas near tectonic plate boundaries.
The second largest geothermal field in the U.S., the Salton Sea in California, has the potential to supply 40% of global lithium demand. Currently looking to produce lithium in the region is Controlled Thermal Resources Ltd. (CTR), a public unlisted energy company developing the Hell’s Kitchen lithium project (Figure 4).
Another example of geothermal brine deposit is found in the Rhine Valley, Germany, where the Australia-based Vulcan Energy Resources (ASX:VUL) aims to develop the first zero-carbon process plant to produce battery-grade lithium hydroxide.
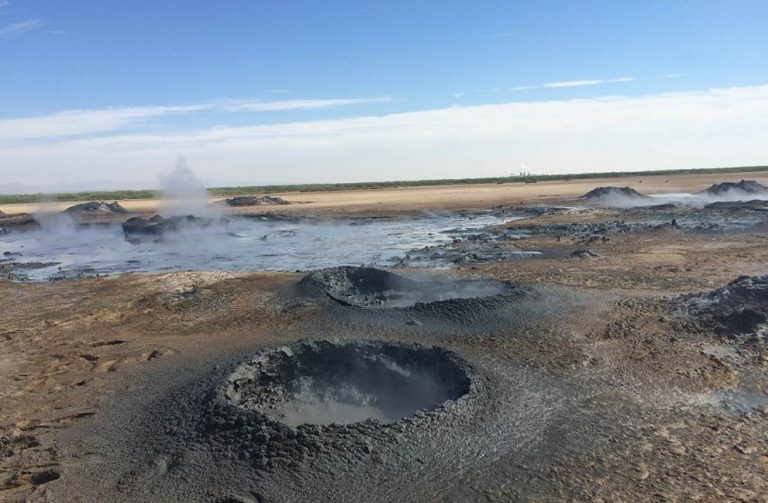
Oilfields
Lithium brine deposits found in deep oil reservoirs account for an additional 3% of known global lithium resources. North Dakota, Wyoming, Oklahoma, Arkansas, and Texas are home to oil field brines with lithium concentration of up to 700 milligram per liter (mg/L). Some of these brines are being developed by Standard Lithium (OTCQX:STLHF, TSX.V:SLL), a Canada-based company focused on the production of lithium from the Smackover Formation in southern Arkansas. The Smackover formation is a porous and permeable limestone sequence that hosts extensive brines and hydrocarbons. Its brines host one of the major bromine assets in the world and significant amounts of lithium (150-500 mg/L). The company’s flagship, the Lanxess project, comprises the largest brine production and processing facilities in North America with resources of 3.14 million tons lithium carbonate equivalent (LCE).
Mineralization
Major controls on the formation of lithium brine deposits include (i) arid climate (ii) closed basin (iii) tectonically driven sagging (iv) associated geothermal/igneous activity (v) favorable source rocks and (vi) enough time to form the brine (Figure 5). Essentially, lithium is either released by weathering or originated from hydrothermal fluids in a closed basin. All lithium-bearing closed basins of current economic interest are Quaternary (from 2.6 million years ago to the present). Brines that can be mined for profit usually have 200-4,000 mg/L lithium. Some elements also available in the brines like boron and potassium can be extracted as byproducts, while other elements can create problems during processing (e.g., magnesium).

Lithium Extraction and Processing
The process of extracting lithium from brine deposits is:
- Lithium-bearing groundwater, typically carrying 200-1,400 milligrams per liter (mg/L) Li, is brought to the surface to evaporate in large basins
- A concentrate of 1-2% Li is obtained after a few months to a year, depending on climate
- The solution is then processed in a chemical plant to yield various end-products, e.g., lithium carbonate and lithium metal.
Mining brine deposits involves extraction of lithium carbonate from the underground brine. This can be done by using solvents that collect lithium ions or membranes. The resulting liquid is then pumped into a plant where it goes through a process of selective absorption via evaporation. The solution is processed in several stages until the lithium is suitable for the sought-after use.
Lithium extraction in geothermal brines uses techniques like nano-filtration or ion exchange resins that act as a chemical sieve to selectively collect only lithium chloride. The latter is then concentrated to produce lithium hydroxide – the end-product used in batteries.
For lithium deposits hosted in oil fields in southern Arkansas, brine is extracted from wells and transported to be processed in bromine-processing plants. Standard Lithium developed a more effective process other than the conventional process of lithium extraction from brines via evaporation called “LiSTR”. The latter uses a crystalline ceramic adsorbent material to selectively extract lithium from the waste brine after it has gone through the bromine-extraction step. Lithium accumulated in the adsorbent material is then released for recovery.
Future Outlook
To keep pace with soaring medium- and long-term demand driven by batteries, lithium production capacity will have to follow suit. Yet, it is important to consider the particularities of different lithium sources (Figure 6). Cost-wise, hard rock mining may seem more expensive than brine mining. However, this is due to the high wages paid in Australia, the main hard rock lithium producer. Some stumbling blocks to brine mining include extended time and large funding required to start production, heterogeneous ore grades, geographic and climate controls, and environmental and geopolitical concerns.

One of the main issues related to mining brine deposits is the environmental impact, particularly water use. Approximately 500,000 gallons of water are required to produce one ton of lithium – the equivalent of 3,500 people’s annual water supply. Brine mining is also beset by social hurdles, like impacts on local air quality and contamination of water supply by toxic chemicals used in the separation process. Despite these concerns, brine deposits have been getting a lot of attention of late with new deposits in development worldwide in locations like Nevada and California.
Further Reading
- A cost comparison: Lithium Brine vs. Hard Rock Exploration (website)
- A Preliminary Deposit Model for Lithium Brines – USGS (pdf)