Drilling, geophysics, and good old-fashioned legwork can tell us a lot about mineral deposits, but sometimes these standard methods aren’t enough. Stable isotope analysis is one of the most advanced tools available to shed light on complicated geological problems.
Introduction
Stable isotopes can be applied to many scientific questions, but in mineral exploration the method is most often used to identify the source of ore-forming fluids. This, in turn, allows deposits to be linked to specific times, events, and processes, aiding in future exploration. Stable isotope analysis is rather technical and is only carried out by companies that are seriously committed to understanding their deposits.
What are Stable Isotopes?
The nucleus of an atom is made of protons and neutrons. Every element has a set number of protons, but the number of neutrons can vary. Atoms with different numbers of neutrons are referred to as isotopes.
Isotopes come in two flavors: stable and radioactive. Radioactive isotopes have too many or too few neutrons to be stable and will eventually rip themselves apart, releasing potentially dangerous radiation as well as forming the basis of geochronology, the science of dating rocks and minerals. Stable isotopes will remain unchanged indefinitely and form most of the matter in the universe.
Oxygen (O), for example, always has 8 protons, but can have either 8, 9, or 10 neutrons: three different stable isotopes. Each of these forms the same molecules, but there is a subtle difference: mass. Each extra neutron makes the atom a little heavier, and since neutrons and protons weigh approximately the same, isotopes are given mass numbers based on the total number of protons and neutrons: O with 8 neutrons is 16O, 9 neutrons in 17O, and 10 neutrons is 18O.
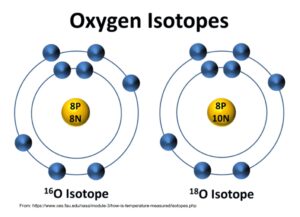
18O is about 11% heavier than 16O, making it harder to move around; if you boil a pot of water the water molecules made with 18O are slightly more likely to remain in the liquid water while the lighter 16O water molecules prefer to form steam. This would leave the steam depleted in 18O relative to 16O (a lower 18O/16O ratio) and the water enriched in 18O/16O. These subtle changes in isotope composition are referred to as fractionation.
How are Stable Isotopes Used?
Many geological events such as metamorphism, igneous activity, and the circulation of hydrothermal fluids can cause isotopes to fractionate. Since these changes are usually very small they are expressed in per mille (‰ or 1/1000) rather than percent (% or 1/100). To help make interpretation simpler, these variations in isotope ratios are expressed relative to an internationally accepted standard, which is assigned a value of 0‰. Samples with higher concentrations of the heavier isotope than the standard will have positive ‰ values while samples with lower concentrations of the heavier isotope will have a negative ‰ value. To remind us we’re talking about a difference in the concentration of a specific heavy isotope, we add the δ symbol followed by the isotope number. In our boiling water example, if the un-boiled water had a δ18O of 0‰, then the boiled water would be 18O enriched and might have a δ18O value of +10 ‰, leaving the 18O depleted steam with a δ18O value of -10 ‰.
Once we have a ‰ value, we can compare it to values of known reservoirs. Seawater, for instance, has a δ18O of 0‰, water released from igneous processes typically has δ18O of +5 to +10‰, and rainwater will have a negative δ18O‰ which varies with latitude. Police and archeologists can even estimate where someone grew up based on the O and hydrogen (H) isotopes in their teeth, which will reflect the ‰ values of the local drinking water.

Stable isotope analysis can be applied to any element that has more than one stable isotope, however fractionation is strongest in lighter elements. 2H, for instance, is twice as heavy as 1H, and H isotopes can fractionate by hundreds of ‰, whereas 65Copper (Cu) is only 3% heavier than 63Cu. Copper rarely fractionates more than a few ‰, a difference that can be very hard to precisely measure. For this reason stable isotope analysis is typically applied to light elements such as H, O, carbon (C), and Sulfur (S) which are found with metals, rather than the metals themselves. Advances in science are enabling heavier metals such as zinc and Cu to be measured, but these are not yet widely used.
Stable isotopes are measured with mass spectrometers, large, expensive machines that require highly qualified personnel to operate; most companies wishing to conduct this work would do it as part of a larger study run by a university or hire an outside lab. Stable isotope analysis is never carried out in isolation, getting accurate and meaningful stable isotope data requires a thorough understanding of the relationships between minerals. Fractionation is heavily influenced by the temperature at which minerals formed, so this at least must be known. Multiple stable isotopes, such as H, O, and C, are often analyzed together to give a more complete picture of how ore formed.
Example: Stable Isotopes in Giant and Not-So-Giant IOCG Deposits
Olympic Dam, Australia, is a world-class Cu-Au-Ag-U deposit with resources of 10982 Mt at 0.73% Cu, 0.24 kg/t U3O8, 0.31 g/t Au, and 1.2 g/t Ag. It didn’t form alone; dozens of other IOCGs formed at about the same place and time, but most have little mineralization to speak of. As well as southern Australia, enormous IOCG breccias also formed at this time in the Wernecke Mountains in present day Yukon, Canada (Canada and Australia were part of the same continent at the time) but contain only very low-grade mineralization. So what’s so special about Olympic Dam?
Most sulfur in Olympic Dam has δ34S values near 0‰, which is consistent with igneous sulfur, but individual sulfur-bearing minerals have a wide range of values (~ -20 to +20‰) suggesting these fluids went through a complex process of reacting with various minerals in the host rocks as well as oxygen in the air. This means that ore-forming fluids at Olympic Dam were sourced from magma but then reacted extensively with a uniquely favorable local environment to produce a uniquely massive deposit.
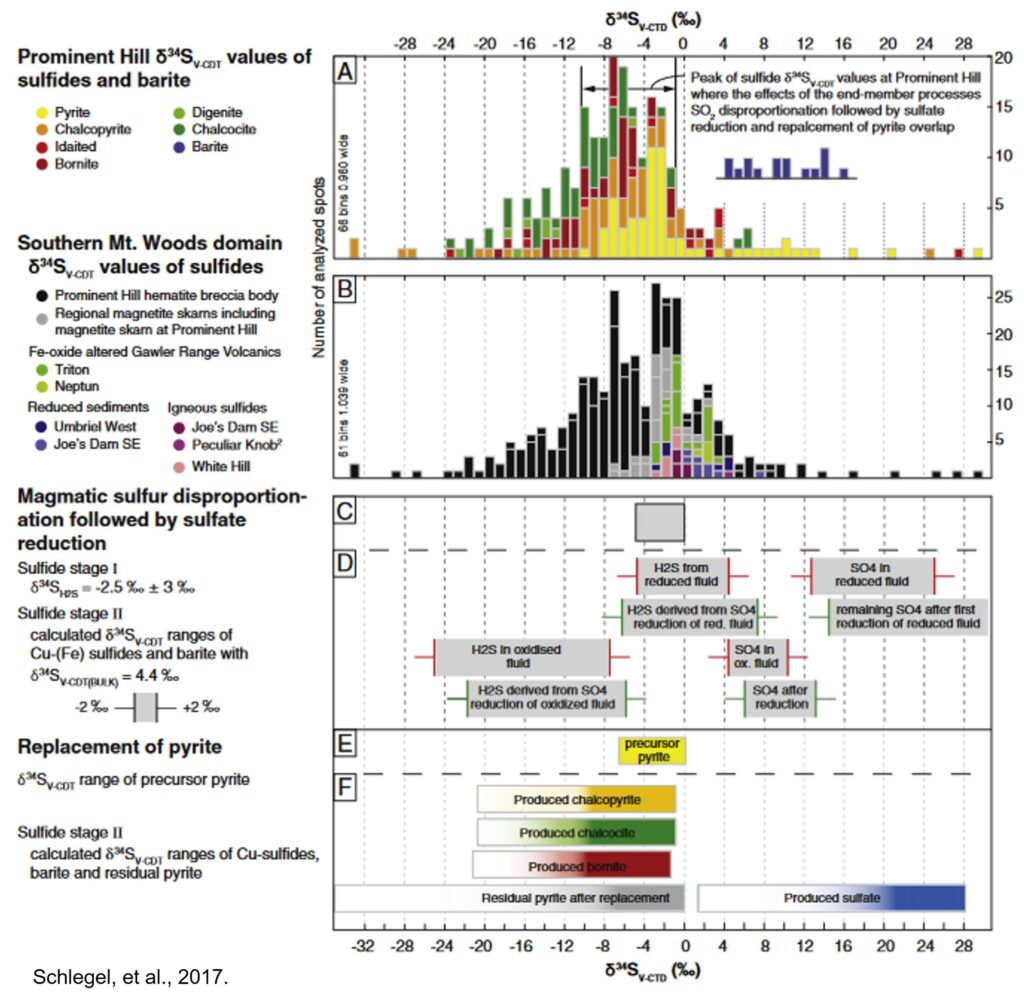
The Wernecke Breccia IOCGs occur sporadically over much of the central Yukon Territory, with some deposits containing slabs of broken rock the size of skyscrapers that’ve been shattered and thrown by a force so vast it was once thought that only an asteroid impact could’ve produced them. Despite their scale, none of these deposits are known to host ore-grade mineralization.
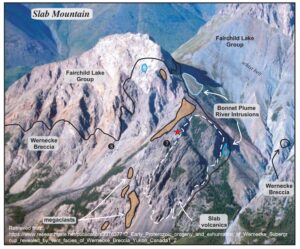
Sulfur isotopes in the Wernecke Breccias range from δ34S of -12 to +17‰, which could be from magmatic, metamorphic, or sedimentary sources. Oxygen and hydrogen isotopes are more revealing: they contain a too high a concentration of heavy O and H to be from magma, but instead seem to be derived from metamorphic fluids mixing with an even heavier source, likely seawater enriched in heavy isotopes through evaporation.

The takeaway: Olympic Dam and the Wernecke Breccias formed at a similar time and place, but through very different processes. There’s unlikely to be an Olympic Dam size deposit in the Wernecke, which may explain what few mining companies are actively exploring this remote region.
Investor Takeaways
Stable isotope analysis is one of the most advanced techniques available to investigate the formation of ore deposits. It is a complicated and technical method which is typically undertaken as part of a detailed study of a deposit’s genesis, and when correctly used it can provide uniquely detailed information on how and why mineralization occurred. Relatively few companies choose to carry out such sophisticated studies, doing so requires significant commitment to a property. Stable isotope analysis can’t find a deposit by itself, instead it is a tool that can point towards where to look, and not to look, when simpler methods aren’t enough.
Further Reading
- Hunt, J. A., Baker, T., Cleverley, J., Davidson, G. J., Fallick, A. E., and Thorkelson, D. J. (2011): Fluid inclusion and stable isotope constraints on the origin of Wernecke Breccia and associated iron oxide – copper – gold mineralization, Yukon. Canadian Journal of Earth Science, 48, 1425-1445. (Academic Article)
- Schlegel, T. U., Wagner, T., Boyce, A., Heinrich, C. A. (2017): A magmatic source of hydrothermal sulfur for the Prominent Hill deposit and associated prospects in the Olympic iron oxide copper-gold (IOCG) province of South Australia. Ore Geology Reviews, 89, 1059-1090. (Academic Article)
Subscribe for Email Updates